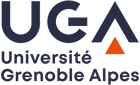
Background
​
From the continuum mechanics point of view, a number of geophysical objects are both (1) damageable solids in which highly localized features and discontinuities emerge as a result of failure and fracturing and (2) materials experiencing large, permanent strains that dissipate stresses. The representation of both types of deformation is necessary to model a number of natural phenomenon such as the drift of sea ice, volcanic eruptions, landslides and lithospheric faulting.
​
My main research interests consist in developing modeling frameworks that can represent a wide range of mechanical behaviours while remaining mechanically simple and numerically efficient and that can allow improving both our physical understanding and prediction capabilities of natural phenomena.
In particular, I am interested in filling the gap between the modeling of the small deformations associated with the damaging/fracturing of geomaterials and the modeling of the much larger deformations that occurs once these materials are highly fragmented and which can lead to catastrophic events.
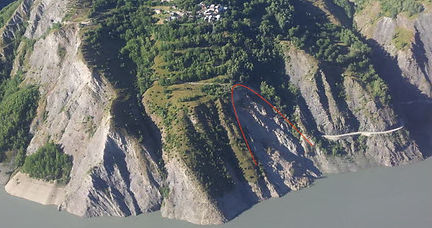
Two examples of natural phenomena involving both small (damaging /fracturing) and large post-damaing deformations. Above: a landslide cutting the road over the Chambon dam (Isère, France). Credit photo: Alain Berthet. Below: Satellite images of an extensive sea ice breakup event off the northern coast of Alaska in February-March 2013. Credit : NASA Earth Observatory.
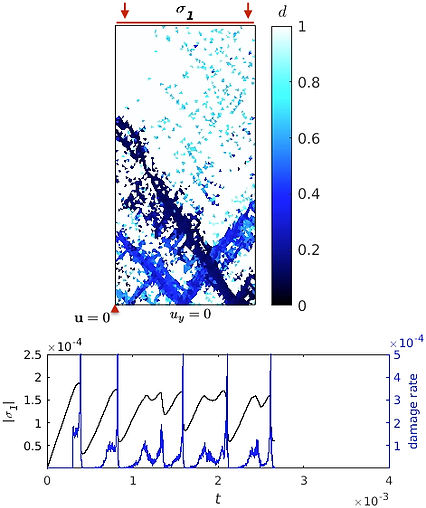
Numerical simulation of the uniaxial compression of a 2D rectangular plate of a brittle material (rock or ice) with the Maxwell-EB model. The plate is compressed by applying a uniform, constant y velocity on the top edge and maintaining the bottom edge fixed in the y direction. The simulation is started from an undamaged plate. The field of the level of damage, d, is shown, which represents the density of cracks in the material at the model element scale (d = 0 for a "completely damaged" ice and d = 1 for undamaged material). The time series (bottom) shows the evolution of the macroscopic compressive stress integrated on the top plate boundary (black curve) and the damage rate (instantaneous change in level of damage integrated over the domain, blue curve). Each cycle of (slow) loading/healing and (sharp) stress relaxation is associated with the formation of a fault/lead of different shape and orientation.
​
WATCH THE VIDEO of the complete uniaxial compression simulation here: https://youtu.be/wMtZq0IfzZI.
Ph.D. thesis
Making reliable predictions of the drift and deformation of the floating ice that covers the polar oceans is becoming essential in the context of (1) forecasting the opening of shipping routes, (2) inferring the mechanical constraints on offshore structures and ships and (3) estimating the future evolution of its volume and extent to evaluate its short to long-term impact on the climate. This thin sheet of ice is a highly complex material. Under the action of the winds and ocean currents, it deforms rapidly, or breaks, in the brittle regime. Much larger deformations occur once faults, or “leads”, are formed that divide the ice cover into individual ice plates, called “floes”, as these plates move relative to each other with much reduced mechanical resistance, setting the overall drift patterns of the ice cover.
​
In sea ice models, both the small deformations leading to the fracturing of the ice cover and the large deformations translating into its drifting motion must be accounted for. The objective of my thesis was to develop a new mechanical modeling framework capable of representing these two types of deformation and that could be easily implemented in the current continuum sea ice models used for regional and global climate predictions. The rheological framework developed draws from the similarity in the mechanical behaviour of sea ice and rock-like materials and combines the concepts of
-
elastic memory,
-
progressive damage mechanics,
-
viscous-like relaxation of stresses,
-
recovery of mechanical strength through healing.
Idealized and realistic simulations have shown that this model, called Maxwell-Elasto-Brittle (Maxwell-EB), reproduces the main characteristics of sea ice mechanics, which are the strain localization, the anisotropy and the intermittency of deformation (Dansereau et al., 2016).
Post-doc research
After my Ph.D., I continued developing the Maxwell-EB rheology and its parameterizations in the view of its implementation into continuum, thermodynamic-dynamic sea ice and ice-ocean coupled models.
The first implementation of the Maxwell-EB model on geophysical scales was made in the context of representing the complex mechanical and dynamical behaviour of sea ice drifting through a narrow passage of the Canadian Arctic (Dansereau et al., 2017). This implementation tested the capability of the mechanical framework to reproduce the formation of stable ice arches (or “bridges”), with flow stoppage upstream and complete detachment of the ice downstream, a phenomenon also commonly observed in other granular cohesive materials (e.g., soil and sand in a silo). The model was also able to reproduce other observed phenomena such as the strong localization of the thickest ice in the so-called “pressure ridges” (linear rubble piles of ice, several meters thick, tens of meters wide and up to several kilometers long) and the persistence of landfast ice along the coast and in fjords. The Maxwell-EB framework has recently been implemented into two dynamic-thermodynamics sea ice models: LIM3 (Nucleus for European Modelling/Louvain-la-Neuve Sea Ice Model) and NeXtSIM.
​
​
Recent research
I recently applied a similar visco-elastic-brittle rheology to the modelling of the deformation of pressurized volcanic edifices. In this case, the addition of a viscous relaxation mechanism for the stresses to an elastic-damageable framework allows accounting for the progressive damage of the edifice as wells as the anelastic deformations that cumulate and lead to rupture and eruption.
​
My work has also been interested in some more fundamental problems of mechanics, such as the relevance of Coulomb's failure theory in representing the failure of brittle materials in elastic damage models and the use of a damage-dependant viscous relaxation of stresses to represent the granular fluid to solid transition in a continuum damage model.
​
I am currently working on multi-scale modelling with an application to the deformation of rocks .
​
​
I am looking forward to further develop these modeling concepts in the context of other problems involving the complex mechanical behaviour of geomaterials.
​
Find my contact information here.
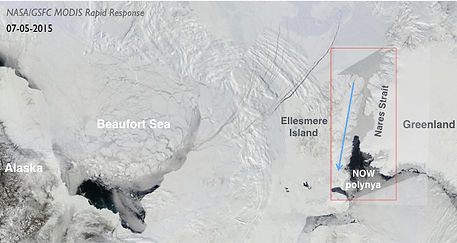
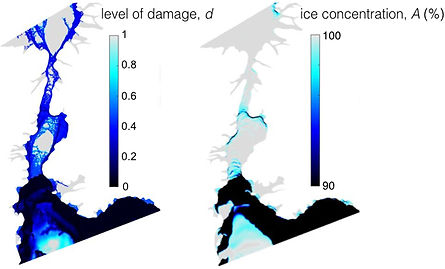
(Top) Moderate Resolution Imaging Spectroradiometer (MODIS) reflectance image showing the state of the ice cover over the Canadian Basin side of the Arctic on May 7, 2015 (http://rapidfire.sci.gsfc.nasa.gov/imagery/). A stable ice bridge is observed in Nares Strait (red rectangle) at the constriction between Kane Basin and Smith Sound and the North Water polynya is open. The superimposed grey shading indicates the coverage of the domain used in the Maxwell-EB simulations.
(Bottom) Instantaneous fields of the (left) level of damage and (right) ice concentration (percentage of ice versus ocean covered portion of a model grid cell) simulated simulated with the Maxwell-Elasto-Brittle model. The simulation is started with a uniform ice concentration (100%) and a uniform 1 m ice thickness. A spatially uniform, northerly (along-channel) with stress is applied. It is increased from 0 to 1 N per meter squared over a period of 24 hours and then held constant. The ocean is at rest and a passive drag is applied on the drifting ice cover. The fields are snapshots obtained after 72 hours of simulation (real time).
WATCH THE VIDEO of the entire simulation here: https://youtu.be/wylcpagTTxA.